Full Text
Introduction
Coronaviruses (CoVs) are the largest group of viruses belonging to family Coronaviridae and the order Nidovirales and can cause respiratory, digestive, and nervous system diseases in humans and many other animals [1]. CoV were thought as weak pathogen for humans causing mild flu-like illness but with consistent outbreaks like severe acute respiratory syndrome (SARS) in 2002, Middle East respiratory syndrome (MERS) in 2012 and now COVID-19 [2]. Emergence of new strains from their zoonotic hosts and repeated transmission leading to global economy losses makes CoV vaccines highly desirable, as presently there are no specific antiviral drugs are available against CoV [3].
COVID-19 has been declared as a Public Health Emergency of International Concern. The elderly and people with underlying diseases are susceptible to infection and prone to serious outcomes, which may be associated with acute respiratory distress syndrome (ARDS) and cytokine storm. Due to the rapid increase of severe acute respiratory syndrome coronavirus 2 (SARS-CoV-2) infections and unavailability of antiviral therapeutic agents, developing an effective SAR-CoV-2 vaccine is urgently required [4-6].
Genome: Coronaviruses contain a non-segmented, positive-sense RNA genome of ~30 kb; 5′ cap structure along with a 3′ poly (A) tail [1, 7], allowing it to act as an mRNA for translation of the replicase polyproteins.genome of SARS-CoV-2 is transcribed in at least 10 open reading frames (ORFs). The replicase gene encodes non-structural proteins (nsps) & structural and accessory proteins. ORF1ab translates into a polyprotein which is processed into 16 non-structural proteins (NSPs). SARS-CoV-2 virus contains four structural proteins namely, spike (S), nucleocapsid (N), envelope (E), and membrane (M) proteins & the organization of the genome is 5′-leader-UTR-replicase-S (Spike)-E (Envelope)-M (Membrane)-N (Nucleocapsid)-3′ UTR-poly (A) tail with accessory genes interspersed within the structural genes at the 3′ end of the genome (Figure 1) [1].
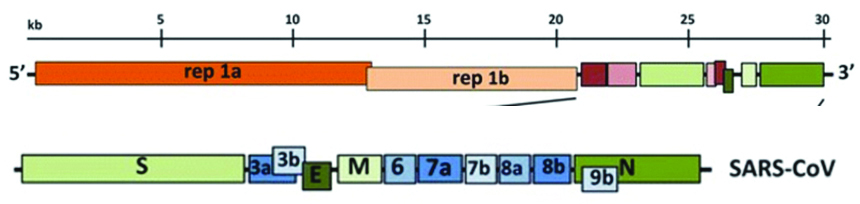
Figure 1: Expanded regions showing structural and accessory proteins in the 3′ regions of the HCoV-229E.
Viral structure is primarily formed by the structural proteins such as spike (S), membrane (M), envelope (E), and nucleocapsid (N) proteins (Figures 2, 3). The S, M, and E proteins are all embedded in the viral envelope, a lipid bilayer derived from the host cell membrane. The N protein interacts with the viral RNA in to the core of the virion.
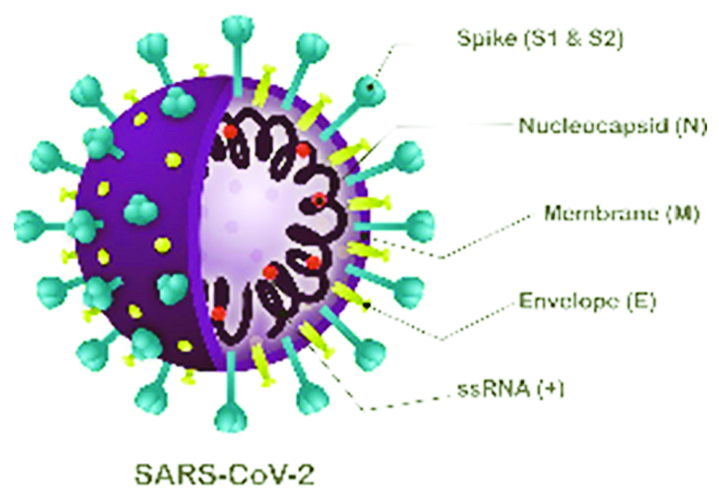
Figure 2: Structural proteins.
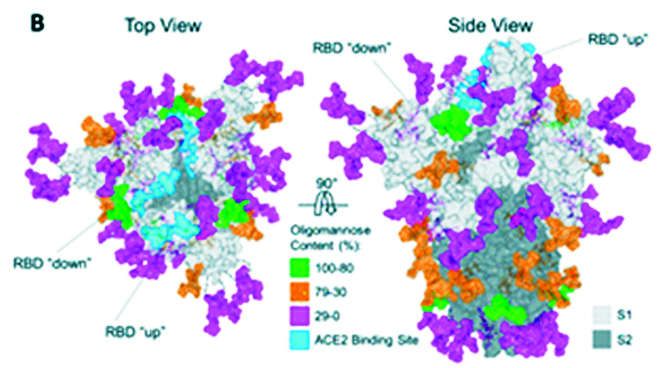
Figure 3: Crystal structure of the S protein.
Spike (S) structural protein [5, 7]
SARS-CoV-2 uses the human angiotensin-converting enzyme 2 (hACE2) receptor to seize the target cell through the spike glycoprotein. S glycoprotein, multi-functional trans-membrane protein, plays vital role of viral attachment, fusion, and entry into the host cell .S protein consists of S1 and S2 subunits. S1 subunit has two functional domains viz. N-terminal Domain (NTD) and Receptor Binding Domain (RBD). S2 subunit has three operational domains namely, fusion peptide (FP), heptad repeat (HR) 1, and 2.
E (envelope) structural protein
E protein is involved in the viral replication cycle involving assembly, release, pathogenesis.
M structural protein
M protein is the central organizer of CoV assembly. It is most abundantly expressed in the virus particle. It functions crucially in the morphogenesis and assembly of the SARS-CoV-2. In case of SARS-CoV, the M protein has also been shown to induce the process of apoptosis in the host cell.
N structural protein
N protein is an antagonist of the antiviral RNAi. It is responsible for the inhibition of the cell cycle of the host cell as it can inhibit the entry of the cell into the S-phase.The binding of the M and N protein stabilizes the N protein and RNA complex, and the internal core of the virus.
Objectives of the COVID vaccine strategy
- Ensuring the quality, safety and efficacy of vaccines
- Securing timely access to vaccines for Member States and their population while leading the global solidarity effort
- Ensuring equitable and affordable access for all to an affordable vaccine as early as possible
Designing a coronavirus vaccine [2, 4, 8, 9]
Ideally, vaccines should provide protection not only against current versions of pathogens but also against those likely to emerge in the future. To meet the urgent need for a vaccine, a new pandemic vaccine development paradigm has been proposed that compresses the development timeline from 10–15 years to 1–2 years.
Vaccine design concerns the selection of antigens, vaccine platforms, and vaccination routes and regimen.
Emerging evidence suggests the requirement of both antibody-mediated and T cell-mediated immunity for effective protection against SARS-CoV-2. A COVID-19 vaccine that can induce trained immunity (Innate immune memory) might enhance early viral control by overcoming virus-imposed innate immune suppression and facilitating adaptive immune activation. The early timing of action by trained immunity is of importance as the overproduction of cytokines by macrophages at later stages of COVID-19 can contribute to immunopathology.
Vaccine platforms [4-6, 10, 11]
Vaccine platforms are divided into six categories (Table 1): live attenuated virus, recombinant viral-vectored vaccines that are bioengineered to express target pathogen antigens in vivo, inactivated or killed virus, protein subunit vaccines, virus-like particles (VLPs) and nucleic acid-based (DNA or mRNA) vaccines (Figures 4, 5) [12].
Table 1: Mechanism of action of various vaccine candidates [4-6, 10, 11].
1
|
Nucleic acid-based vaccines
|
DNA Vaccine
|
Plasmid DNA(S, M and N genes) encoding protein from SARS CoV 2
|
Induce both T(Cell Mediated Immune (CMI) response) and B(humoral) cell responses
|
|
|
mRNA vaccines
|
Synthetic mRNA encapsulated in Lipid nanoparticle (LNP) which codes for the full-length, pre-fusion stabilized spike protein (S)
|
Humoralimmune response
|
2
|
Recombinant vector vaccines
|
use a virus to deliver coronavirus genes into cells
|
CellMediated Immune (CMI) response
|
3
|
Protein-Based Vaccines
|
Use a coronavirus protein or a protein fragment to provoke an immune response
|
CellMediated Immune (CMI) response
|
4
|
Whole-Virus Vaccines(Inactivated virus vaccines)
|
Vaccines that use weakened or inactivated viruses to provoke an immune response
|
Cell Mediated Immune (CMI) response
|
5
|
Repurposed Vaccines
|
Vaccines already in use for other diseases that may also protect against Covid-19.
|
CellMediated Immune (CMI) response
|
6
|
Virus-like particle
|
|
CellMediated Immune (CMI) response
|
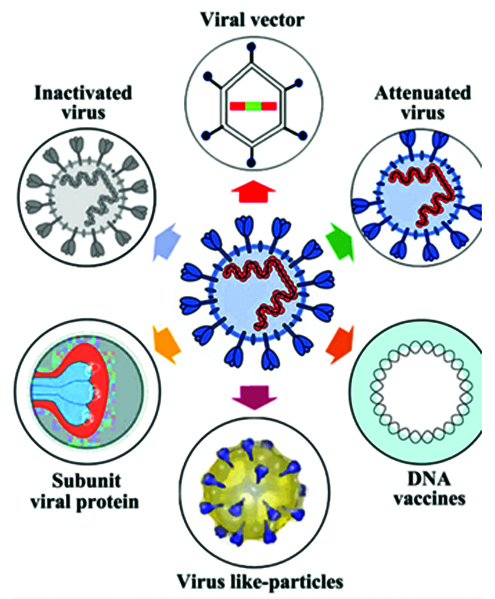
Figure 4: Summary of stratergy types for COVID 19 vaccine development.
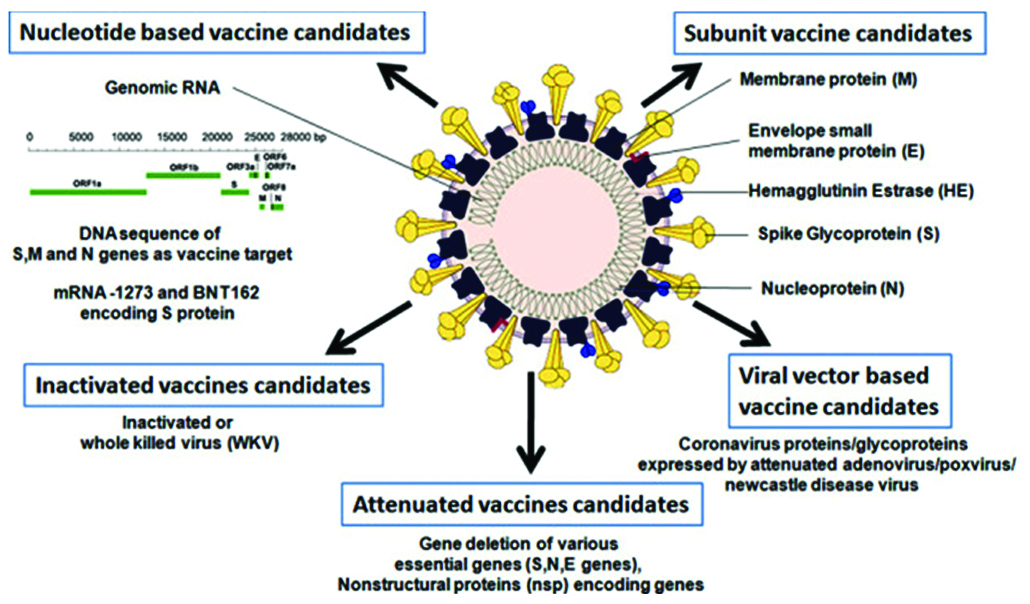
Figure 5: Graphical abstract of candidate vaccines.
Development of the “ideal” vaccine for COVID-19 [13-15]
Research and Development (R&D) involving platform selection (Table 2), designing targets and preclinical testing (Figure 6).
Table 2: Stages of development cycle of a vaccine.
Stage
|
Description
|
Preclinical testing
|
Vaccine tested on cells & experimented on animals such as mice or monkeys to see if it produces an immune response.
|
Phase 1 safety:
|
Vaccine given to a small number of people to test safety and dosage as well as to confirm that it stimulates the immune system
|
Phase 2 expanded trials
|
Vaccine given to hundreds of people split into groups, such as children and the elderly, to see if the vaccine acts differently in them
|
Phase 3 efficacy trials
|
Vaccine given to thousands of people to see how many become infected, compared with volunteers who received a placebo
|
Early or limited approval
|
Vaccine approval without waiting for the results of phase 3 trials (emergency use authorization EUA)
|
Approval
|
Formal approval after ensuring safe and effective
|
Combined phases
|
To accelerate vaccine development combine phases(Phase 1/2 trials)
|
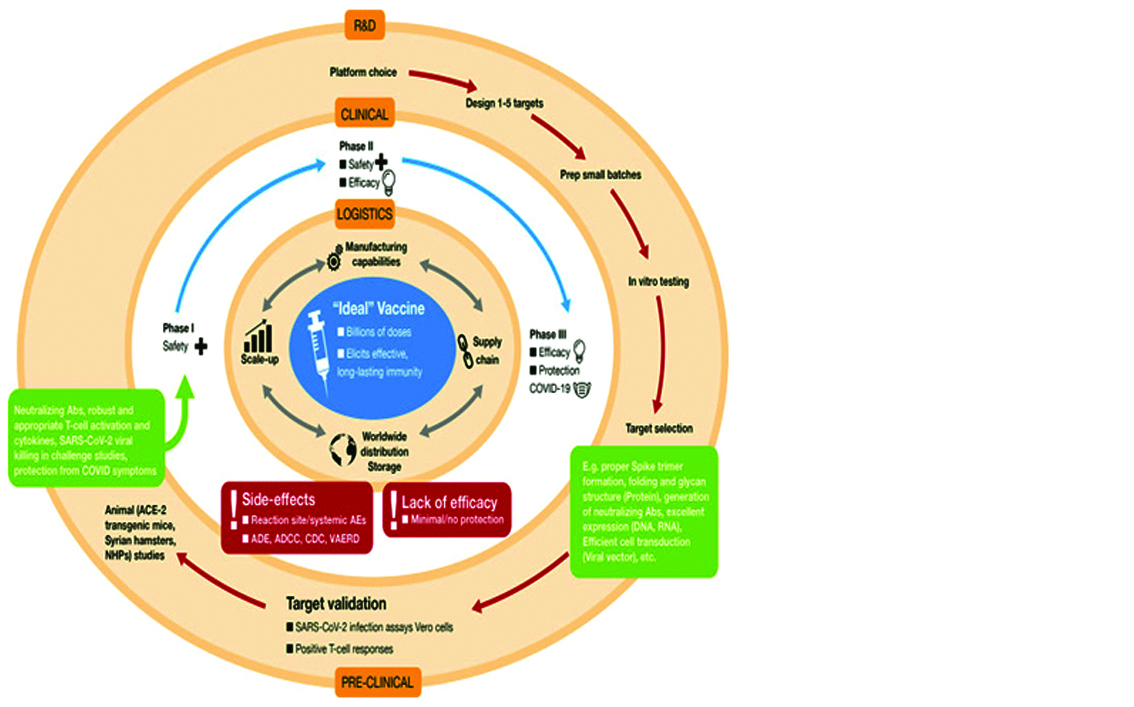
Figure 6: Development of “ideal” vaccine for COVID-19 depicted by concentric circles in three discrete phase.
Clinical trials: Candidate vaccine is taken to the second phase, which consists of testing in human volunteers in three stages of clinical trials (Phase I, Phase II, and Phase III).
Logistics: If vaccine safety and efficacy is achieved in human volunteers, the logistical operations ensure worldwide distribution in a coordinated and inter-connected manner (manufacturing, supply chain distribution, storage, etc.).
Vaccine candidates that do not achieve satisfactory results in clinical trials, due to various factors shown in the red boxes, is dropped from further development. Figure 7 shows the number of candidate vaccines that are currently under clinical and preclinical development.
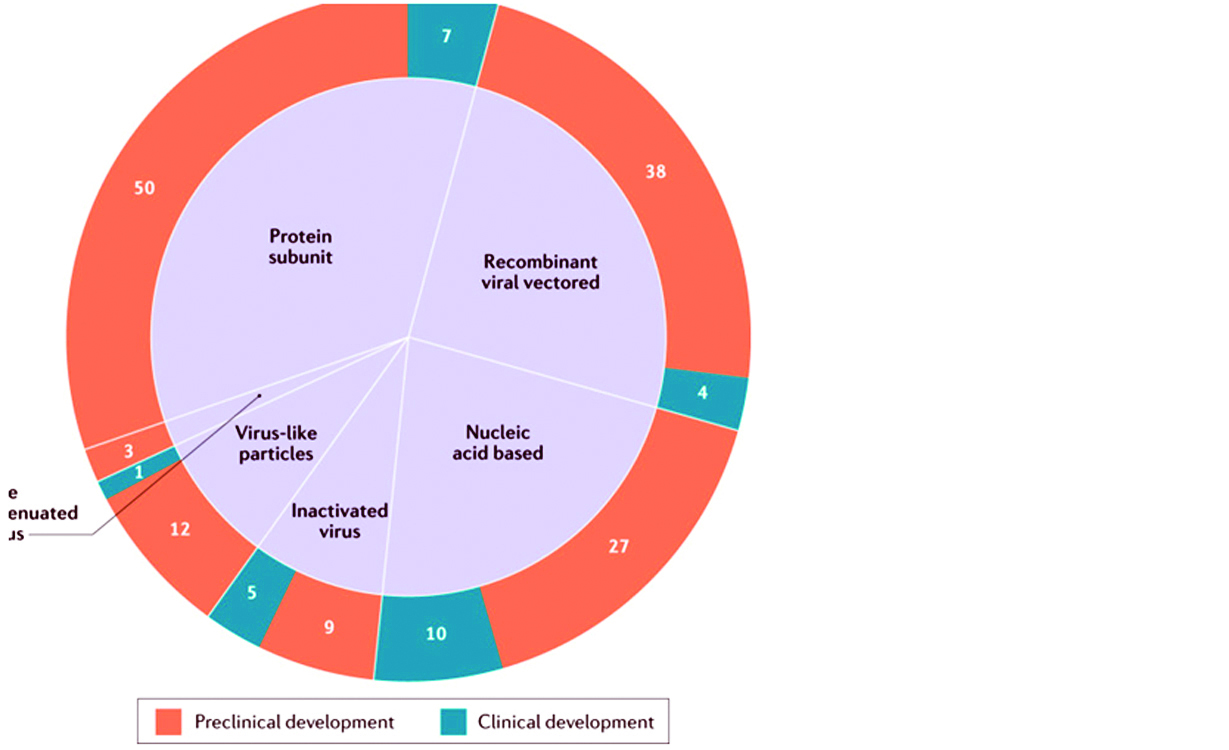
Figure 7: List of candidate vaccines for SARS-COV: Global COVID 19 vaccine landscape showing the number of candidate vaccines that are currently under clinical and preclinical development.
Vaccine-mediated adverse events [5, 6, 14]
According to WHO, “Vaccine must provide a highly favorable benefit-risk contour; with high efficacy, only mild or transient adverse effects and no serious ailments”. Creating herd immunity against SARS-CoV-2 may require a vaccine efficacy rate of only ∼70% if the basic reproductive number for the infection in a naive population is ∼3 [5].
Areas of concern related to vaccine administration include: ADE: antibody-dependent enhancement; ADCC: antibody-dependent cellular cytotoxicity; CDC: complement-dependent cellular cytotoxicity; VAERD: vaccine-associated enhanced respiratory disease.
Front-runner vaccines include Sino Biotech’s CoronaVac, which is an inactivated virus vaccine; Moderna’s mRNA1273; Johnson & Johnson’s JNJ-78436735, which is an adenovirus-based vaccine; Pfizer’s BNT162b2, which is an mRNA-based vaccine; the University of Oxford’s candidate ChAdOx1 nCoV-19, which is an adenovirus-based vaccine; Sinovac’s SARS-CoV-2 vaccine, which is an inactivated candidate; CanSino’s Ad5-nCoV, which is a viral vector vaccine; Russian Gamaleya Institute’s Sputnik V, which is an adenovirus-based vaccine. Rapidly progressing anti COVID-19 vaccines are listed in Table 3.
Table 3: Rapidly progressing anti COVID-19 vaccines [8, 11, 15].
S.no.
|
Type of vaccine
|
Developer
|
Clinical trial stage
|
Platform :Viral vectored vaccines
|
1
|
Adenovirus Type 5 vector/ Non-replicating viral vaccine/ Ebola
|
CanSino Biological Inc./ Beijing Institute of Bio-technology
|
Phase 2
|
2
|
Inactivated viral vaccine/ Inactivated
|
Wuhan Institute of Biological Products/ Sinopharm
|
Phase 1/2
|
3
|
Lentiviral based minigene dendritic cell (DC) and T cell vaccine (LV-SMENP-DC)
|
Shenzhen Geno-Immune Medical Institute
|
Phase 1
|
4
|
The COVID-19/ aAPCs: Pathogen-specific artificial antigen presenting cells (aAPC)
|
Shenzhen Geno-Immune Medical Institute
|
Phase 1
|
5
|
ChAdOx1/ Non-replicating viral vector/
MERS, influenza, TB, chikungunya, zika, MenB, plague
|
University of Oxford/ AstraZeneca
|
Phase 3
|
6
|
Inactivated (formaldehyde inactivated + alum)/ SARS
|
Sinovac
|
Phase I/II:
|
7
|
Gam-Covid-Vac/ SputnikV adeno-based Gam-COVID-Vac/ Non-replicating viral vector
|
Gamaleya Research Institute
|
Phase 3
|
8
|
Ad26 (alone or with modified vaccinia virus ankara {MVA} boost), Non-replicating viral vaccine/ Ebola, HIV, RSV
|
Janssen Pharmaceutical Companies/ Beth Israel Deaconess Medical Center
|
Pre-Clinical (Phase 1 in September 2020)
|
9
|
Influenza vector expressing RBD: DelNS1-SARS-CoV2-RBD/ Replicating viral vector (LAV)/ MERS
|
University of Hong Kong
|
Pre-clinical
|
Platform: Inactivated whole-virus vaccines
|
1.
|
Covaxin
|
Bharat Biotech + Indian Council of Medical Research and NIV Pune
|
Phase 2/3
|
Platform: DNA vaccines
|
1
|
DNA Plasmid Vaccine (INO-4800)/ Lassa virus, Nipah virus, HPV, HIV, Filovirus
|
Inovio Pharmaceuticals
|
Phase 1
|
2
|
Electroporated linear DNA vaccine
|
LineaRx | Takis Biotech
|
Pre-clinical
|
3
|
Electroporated DNA vaccine
|
ZydusCadila
|
Pre-clinical
|
Platform: RNA vaccines
|
1
|
LNP- Encapsulated mRNA (mRNA-1273)
|
Moderna/NIAID
|
Phase 2
|
2
|
CureVac mRNA/
RABV, LASV, YFV, MERS, InfA, ZIKV, DengV,
|
CureVac
|
Phase 1
|
3
|
LNP-nCoVsaRNA/ RNA/ EBOV, LASV, YFV, MERS, InfA, ZIKV, DENV, NIPV)
|
Imperial College London
|
Phase 1
|
4
|
BNT162/ mRNA
|
BioNTech| FosunPharma| Pfizer
|
Phase 1 /2:
|
4
|
LNP-encapsulated mRNA cocktail encoding VLP/ RNA
|
Fudan University/ Shanghai JiaoTong University/RNA Cure Biopharma
|
Pre-Clinical
|
Platform: Protein subunit vaccine
|
1
|
VLP recombinant sub-unit, full length S trimer/ nanoparticle + Matrix M (NVX-CoV2373)/RSV)
|
Novavax| Emergent Bio Solutions
|
Phase 1:
|
2
|
Molecular Clamp Stabilized Recombinant spike protein/Subunit/
Nipah, influenza, Ebola, Lassa
|
University of Queensland | GSK| Dynavax
|
Pre-clinical
|
3
|
Recombinant protein subunit vaccine/ Influenza, SARS-CoV
|
Sanofi
|
Pre-clinical
|
Platform: Virus like particle (VLP) vaccine
|
|
Virus like particle (VLP) vaccine/
|
Medigaco
|
Pre-clinical
|
Platform: Live attenuated vaccine
|
1
|
Deoptimized live attenuated virus/ HAV, InfA, ZIKV, FMD, SIV, RSV, DENV /)
|
Codagenix/ Serum Institute of India
|
Pre-clinical
|
2
|
TNX-1800, live attenuated horsepox virus/ smallpox, monkeypox)
|
Tonix Pharmaceuticals
|
Pre-IND
|
3
|
Live attenuated recombinant measles virus (rMV
|
ZydusCadila
|
Pre-clinical
|
CCHF: Crimean-Congo Hemorrhagic Fever; CHIKV: Chikungunya Virus; DengV: Dengue Virus; FMD: Foot and Mouth Disease; EBOV: Ebola Virus; HAV: Hepatitis A Virus; HBV: Hepatitis B Virus; HIV: Human Immunodeficiency Virus; HPV: Human Papilloma Virus; Inf: Influenza; LASV: Lassa Fever Virus; MenB: Meningitis B; NIPV: Nipah Virus; NORV: Norovirus; RABV: Rabies Virus; RVF: Rift Valley Fever; SARS: Severe Acute Respiratory Syndrome; SIV: Simian Immunodeficiency Virus; TB: Tuberculosis; VEE: Venezuelan Equine; Encephalitis Virus; VZV: Varicella Vaccine (Chickenpox); YFV: Yellow Fever Virus; ZIKV: Zika Virus; Viral vector vaccines: combination of two adenoviruses, Ad5 and Ad26, both engineered with a coronavirus gene.
Safety and efficacy of COVID-19 vaccine
Safety and efficacy are significantly dependent on the type of vaccine, i.e., the technology or platform used. Global immune deficiency is a risk factor for anti-COVID-19 vaccine efficacy, particularly in elderly who have been exposed to a myriad of factors that contribute to weakening of the immune system. Mechanistic reasons for these diseases include weakness of antigen recognition, decreased immune cell quantity and functionality, increased level/ length and timing of humoral immune alterations of components, reduced initiation of cellular responses, and memory cell disorders [12].
Conclusions
Development and distribution of an effective and safe vaccine against the coronavirus represents a lasting solution to the COVID-19 pandemic. Ideal vaccine should elicit long-term antigen-specific antibody responses by plasma cells in addition to the development of persisting T cell and B cell memory. Effective strategy till vaccine development include social distancing, efforts to implement immune-enhancing treatments and efforts to identify tactical repurposed treatments, should be considered major public health priorities.
Conflicts of interest
Authors declare no conflicts of interest.
References
[1] Fehr A.R., Perlman S. Coronaviruses: an overview of their replication and pathogenesis. Methods Mol. Biol. 2015; 1282:1–23.
[2] Chen W, Strych U, Hotez PJ. Bottazzi ME. The SARS-CoV-2 Vaccine Pipeline: an Overview. Curr Trop Med Rep. 2020; 1–4.
[3] Tripathi D, Yi G, Vankayalapati R. COVID-19: An update on vaccine development. Indian J Rheumatol 2020; 15(2):70–72.
[4] Amanat F, Krammer F. SARS-CoV-2 Vaccines: Status Report. Immunity. 2020; 52(4):583–589.
[5] Moore JP, Klasse PJ. SARS-CoV-2 Vaccines:‘Warp Speed’ Needs Mind Melds not Warped Minds. J Virol. 2020; 94(17):e01083-20.
[6] Funk CD, Laferriere C, Ardakani A. A Snapshot of the Global Race for Vaccines Targeting SARS-CoV-2 and the COVID-19 Pandemic. Front Pharmacol. 2020; 11:937.
[7] Perlman S, Netland J. Coronaviruses post-SARS: update on replication and pathogenesis. Nat Rev Microbiol. 2009; 7(6):439–450.
[8] Pandey SC, Pande V, Sati D, Upreti S, Samant M. Vaccination strategies to combat novel corona virus SARS-CoV-2. Life Sci. 2020; 256:117956.
[9] Kaur SP, Gupta V. COVID-19 Vaccine: A comprehensive status report. Virus Res. 2020; 288:198114.
[10] World Health Organization Advisory Group on Human Challenge Studies. Feasibility, potential value and limitations of establishing a closely monitored challenge model of experimental COVID-19 infection and illness in healthy young adult volunteers. Geneva: World Health Organization, 2020. Available from: https://www.who.int/publications/m/item/feasibility-potential-value-and-limitations-of-establishing-a-closely-monitored-challenge-model-of-experimental-covid-19-infection-and-illness-in-healthy-young-adult-volunteers. opens in new tab
[11] Jeyanathan M, Afkhami S, Smaill F, Miller MS, Lichty BD, et al. Immunological considerations for COVID-19 vaccine strategies. Nat Rev Immunol. 2020; 20(10):615–632.
[12] Calina D, Docea AO, Petrakis D, Egorov AM, Ishmukhametov AA, et al. Towards effective COVID‑19 vaccines: Updates, perspectives and challenges (Review). Int J Mol Med. 2020; 46(1):3–16.
[13] Funk CD, Laferrière C, Ardakani A. a snapshot of the global race for vaccines targeting SARS-CoV-2 and the COVID-19 pandemic. Front Pharmacol. 2020; 11:937.
[14] Graham BS. Rapid COVID-19 vaccine development. Science. 2020; 368(6494):945–946. [15] Detmer WM, Supported by the Unbound Medicine Team. Coronavirus COVID-19 Vaccines. Updated: November 23, 2020. Available from: https://relief.unboundmedicine.com/relief/view/Coronavirus-Guidelines/2355056/all/Coronavirus_COVID_19_Vaccines