Full Text
Introduction
Accurate determination of the initial severity of the primary brain damage after severe head injury is crucial in establishing neurologic prognosis and to balance the risks and benefits of treatment [1]. Outcome prediction remains difficult because neurologic assessment is often influenced by the usage of sedatives, analgesics, or muscle relaxants. Therefore, biologic markers that can reliably reflect the extent of brain damage while also being easy to measure (i.e., in peripheral blood) have long been searched for.
Previous studies have identified inflammatory response molecules, excitotoxic amino acids, oxidative stress markers, and enzymes in CSF [2-6]. But measurement of CSF biomarkers requires placement of ventricular catheters, which may influence CSF protein levels [7, 8]. Advances in the analysis of brain specific proteins revealed elevated levels of serum S100B and neuron specific enolase (NSE) after stroke, traumatic brain injury, cardiopulmonary bypass surgery, and cardiac arrest [5, 8-12].
S100B is a Ca2-binding protein of astrocytes, oligodendrocytes, and Schwann cells. Serum S100b levels correlate with contusion volume, presence of subarachnoid hemorrhage on CT, and clinical outcome after severe traumatic brain injury [13, 14].
Glial fibrillary acidic protein (GFAP) is a monomeric intermediate filament protein of astrocytes. CSF concentrations of GFAP are elevated in normal pressure hydrocephalus, dementia, and stroke. In patients who died from traumatic brain injury, increased immunoreactivity was present adjacent to cortical contusion zones [9]. GFAP can be measured in peripheral blood [15, 16].
The objective of this study was to determine the predictive value of serum concentrations of the brain-specific proteins S100B and GFAP for clinical outcome at 6 months after severe traumatic brain injury. To determine if S100B & GFAP concentration in the serum are potential outcome predictors of traumatic brain injury.
Methods
Approval of this study was obtained from the institutional ethics committee. Forty-nine patients with severe closed head injury admitted within 24h after the accident to the tertiary Medical Center included. Written informed consent was obtained from a next of kin because all eligible patients were in coma. Exclusion criteria were no blood sample taken, no informed consent, and no possibility of long-term follow up (for a period of 6 months). Severe traumatic brain injury was defined as a Glasgow Coma Score (GCS) of 8 or less following resuscitation. Patients had an immediate CT scan taken after resuscitation. Overall injury severity was measured using the Injury Severity Score (ISS) [17]. The CT scans of all patients were classified by one investigator according to the Trauma Coma Databank (TCDB) criteria [17]. The CT-TCDB classification utilizes the status of the mesencephalic cisterns, the degree of midline shift in millimeters, and the presence or absence of intracerebral or extracerebral hematoma to define six categories. Four categories indicate diffuse injury of increasing severity whereas two categories indicate the presence of a mass lesion. In category I, no visible intracranial pathology is present; in II, cisterns are present with a midline shift of 0 to 5 mm or lesion densities with a volume not exceeding 25 mL; in category III, cisterns are compressed or absent, with a midline shift of 0 to 5 mm, no high or mixed density lesions 25 mL; category IV is the same as cateogry III but with a midline shift of 5 mm; category V is presence of any mass lesion that is surgically evacuated; category VI denotes the presence of any mass lesion 25 mL that is not surgically evacuated.
Treatment of patients, according to international guidelines, was targeted at normal intracranial pressure and maintaining cerebral perfusion pressure [17]. The following data were recorded: age, sex, GCS, pupillary reactions, ISS, CT-TCDB classification, the occurrence of hypoxia (defined as SpO2 < 90%), and hypotension (defined as a systolic blood pressure 90 mm Hg). The outcome was assessed (blinded for initial serum levels) using the Glasgow Outcome Score (GOS) [14] at 6 months, by one rater during the regular patient visits to the clinic.
Analysis of S100b, and GFAP
Venous blood samples were taken upon hospital admission. Blood could clot and, after centrifugation within 30 minutes (1,000 g, 10 minutes), serum was stored at 70oC until analysis.
Protein GFAP & S-100B was analyzed using a commercially available monoclonal two-site immunoluminometric assay (Sangtec Medical, Dietzenbach, Germany) according to the manufacturer’s instructions by using a Liaison automated analyzer (Byk Sangtec, Dietzenbach) [18].
Statistical analysis
Statistical analysis was performed with the Statistical Package for the Social Sciences (SPSS for Windows, version 14.0, Chicago, IL) and the commercial programs Stata (Ver 9) and StatXact (Ver). Pearson’s correlation test was used to test correlation of two variable. Mann–Whitney test was used to test significance between two groups. Logistic regression model was used to evaluate the prognostic ability of biomarkers separately to predict the clinical outcome.
Results
The clinical and demographic characteristics, neuroradiologic data, and time to sample withdrawal of the 49 patients included in this study are summarized in Table 1.
Age
Mean age of presentation was 43.82 years (range 18 – 75 years); of which patients aged more 60 years were five (10.20%). Sex: of 49 patients enrolled there were 41 males (83.7%) and 08 (16.3%) female patients.
Mode of injury
Main mode of injury was road traffic accident in 42 patients (85.7%), followed by fall in six patients (12.2%) and assault in one case (2.0%).
Pupillary reaction
32 (65.3) patients showed equal papillary reaction and in 27 (34.7%) patients showed one or both absent pupillary reaction.
Hypotension: of 49 patients, only six patients (12.2%) had hypotension at admission.
Hypoxia: of 49 patients, 18 patients had hypoxia (36.7%) at admission. Alcohol in toxification: 15 (30.61%) patients consumed alcohol, and history not available six (12.24%) and rest 28 (57.14%) patients have not consumed. Neurological status: Mean GCS score was 7.27 (range 3-12), 16 (32.65%) patients suffered from moderate head injury and 33 (67.34%) severe head injury.
Cranial computed tomography
Initial cranial computed tomography (CCT) finding with regard to clinical outcome are shown in Table 1. No visible intracranial pathology was found in one patient. Diffuse injury was noted in 26 (53.06 %) cases and mass lesion were noted in 23 (46.93%) cases. Evidence of brain stem injury was noted in two cases, of which one of them died 2 months post injury. Because of the small number of brain stem injuries detected in this study, this criterion was not analyzed for its predictive value.
Table 1: Demographics of 49 patients with traumatic brain injury.
Age median (Range)
|
43.82 (18-75)
|
Sex n(%)
Men
Women
|
41(83.70)
08(16.30)
|
Type of accident n(%)
RTA
Falls
Assault
|
42(85.70)
06(12.20)
01(2.0)
|
GCS median (range )
|
7.27(3-12)
|
Pupillary Reaction n(%)
Present
One or both absent
|
32(65.30)
27(34.70)
|
ISS median (Range)
Poly Trauma (ISS>16)
|
09-36
33(67.34%)
|
Hypotension n(%) (SBP<90mmhg)
|
06(12.20)
|
Hypoxia n(%) (spo2<90%)
|
18(36.70)
|
Alcohol intoxication n(%)
No
Yes
Unknown
|
28(57.14)
15(30.61)
06(12.24)
|
CT-TCDBClassification n(%)
Diffuse
Mass
No intracranial
|
26(53.06)
23(46.93)
01(2.04)
|
GOS 6 month n(%) –
Poor outcome
1
2
3
Good outcome
4
5
|
11(22.44)
03(06.12)
06(12.24)
04(08.16)
25(51.02)
|
S100B
On admission, we found mean S100b levels of 270.97 pg/ml (0-1116.9pg /ml). S100b levels after could not be detected in normal healthy individuals. Mean S100b levels were higher in male, aged more than sixty years, absent pupillary reaction group, hypotensive, and hypoxic, and in patients with mass lesion on CCT. Mean value of S100B levels where higher in severe head injury compared to moderate head injury (Figure 1).
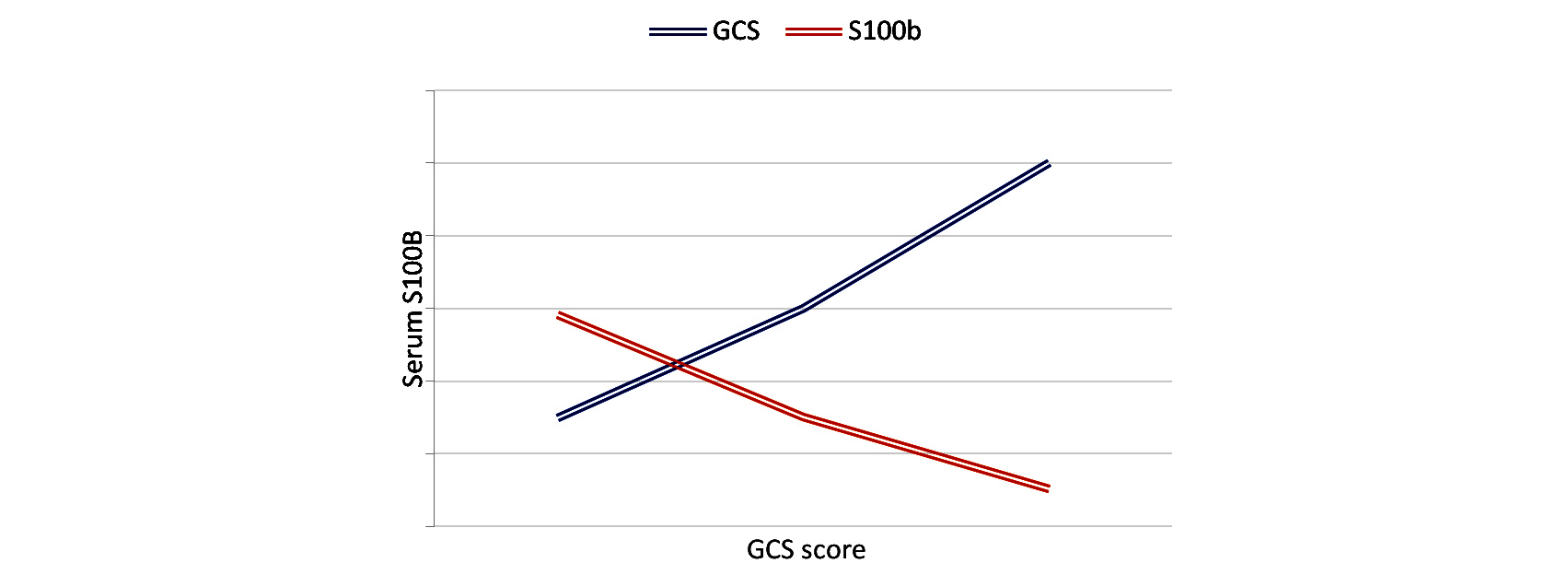
Figure 1: Graph comparing Glasgow coma scale (GCS) Vs S100B level.
Mean value of S100b more in poly trauma patients (ISS > 16) (isolated 112.44pg/ml, polytrauma 358.01pg/ml) analyzed in graph no. ISS score was significantly correlated with S100b serum levels (r: 0.346, P: 0.015) (Table 2).
Table 2: Injury severity score with biomarkers.
Injury severity score
|
S100B
|
GFAP
|
r: 0.346
P:0.015
|
r:0.164
P:0.259
|
CCT does not show any correlation with S100b levels (r: 0.353, p: 0.13). But when a comparison is made between diffuse and mass lesion, mean S100B values were higher in mass lesion compared to diffuse lesion and it was not statically significant (P: 0.298) (Table 3). Means serum concentration of s100b levels is significantly higher in dead patients compared to alive patients (Table 4). Means serum concentration of s100b levels significantly higher in unfavorable patients compared to favorable patients (Table 5).
Table 3: biomarker comparsion between diffuse & mass CCT-scan lesion.
CCT scan
|
S100b
|
GFAP
|
Diffuse
|
227.12
|
2.47
|
Mass
|
320.47
|
6.60
|
P value ( t-test)
|
0.298
|
0.012
|
Table 4: Mean serum level in dead vs alive.
GOS
|
Mean
S100B
|
Mean
GFAP
|
Mann Whitney test
|
|
Dead
|
559.65
|
10.19
|
S1OOB
|
GFAP
|
|
P:0.014
|
P:0.002
|
|
Alive
|
187.36
|
2.73
|
|
Table 5: Mean serum level in unfavorable vs favorable.
GOS
|
S100B
Pg/ml
|
GFAP
ng/ml
|
Mann Whitney test
S100B
|
Mann Whitney test
GFAP
|
Favorable
|
70.66
|
2.59
|
P:0.002
|
P:0.005
|
Unfavorable
|
439.20
|
6.15
|
GFAP
On admission, we found mean GFAP levels of 4.40 ng/ml (0-12.18 g/ml). GFAP levels could not be detected in normal healthy individuals. Mean GFAP levels were higher in male, aged more than sixty years, absent pupillary reaction group, hypotensive and hypoxic patients, severe head injury (Figure 2), and in patients with mass lesion on CCT.
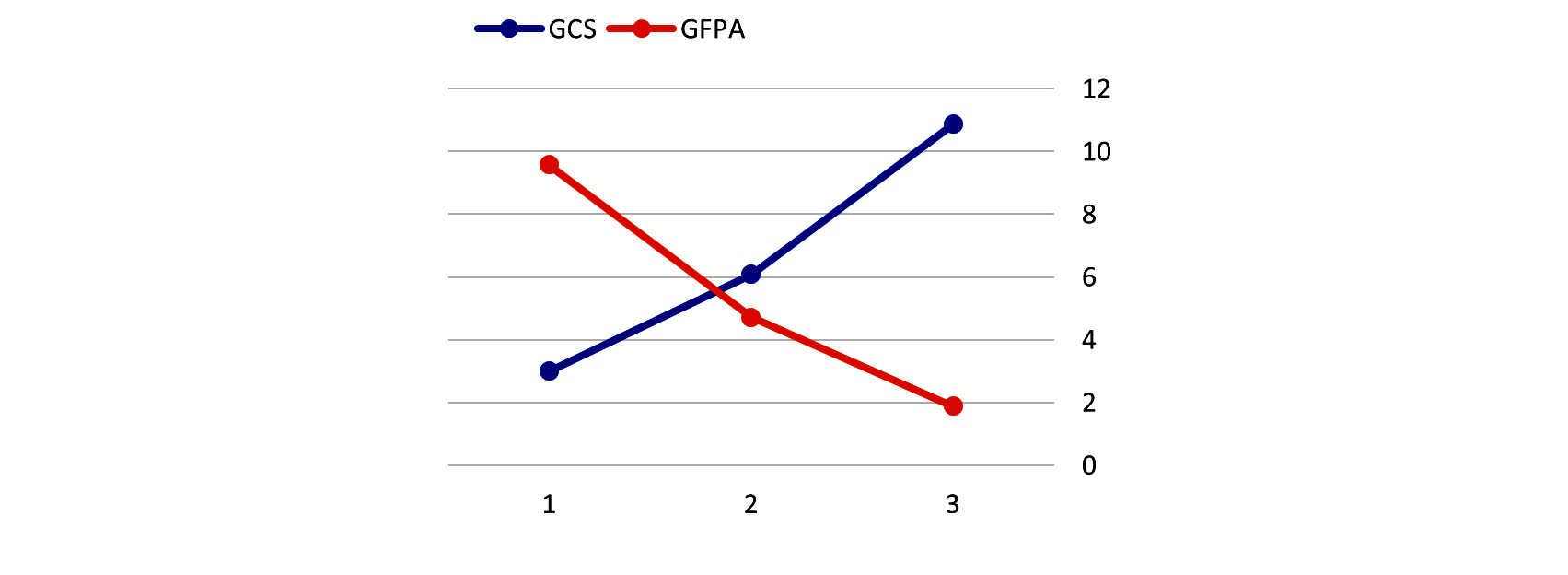
Figure 2: Graph comparing Glasgow coma scale (GCS) Vs Glial fibrillary acid protein (GFAP) level.
Mean value of GFAP more in poly trauma patients (ISS > 16) (isolated 2.53ng/ml, poly trauma 5.53ng/ml) analyzed in graph no. ISS score was not correlated with GFAP serum levels (r: 0.164, P: 0.259) (Table 2). CCT showed significant correlation with GFAP levels (r: 0.555, p: 0.00). Mean GFAP values were observed to be higher in mass lesion when compared to diffuse lesion and it was statically significant (P: 0.000) (Table 3). Means serum concentration of GFAP levels significantly higher in dead patients when compared to alive patients (Table 4). Means serum concentration of GFAP levels significantly higher in unfavorable patients when compared to favorable patients (Table 5).
Clinical outcome
After 6 months, 11 patients died (GOS 1), 3 patients remained in vegetative state (GOS2), 6 patients were severely disabled (GOS3), 4 patients were moderately disabled (GOS4) and 25 patients showed a good recovery. Therefore, 20 patients were categorized to have unfavorable outcome (GOS1-3), and 29 patients showed a good outcome (GOS4-5).
Outcome prediction
The statistical correlation of neurological status on admission, CCT findings on admission, S100b, GFAP levels with clinical outcome are shown in the table. Age, hypotension, hypoxia did not show any significant effect on the outcome (P>0.05). Neurological status on admission showed positive correlation (P :< 0.05). S100b, GFAP, and CCT showed negative correlation (P :< 0.05) with GOS 6th month post injury.
To know the effect of S100b and GFAP in predicting outcome of traumatic brain injury, Univariate Logistic Regression was applied. It was found that both S100B and GFAP are able to significantly predict the clinical outcome following traumatic brain injury (P:<0.05). When multivariate logistic regression was applied, only S100 was found to be significant (P: 0.009) (Table 6).
Table 6: Outcome prediction of gcs, cct, S100b, GFAP.
Variable
|
Correlation
P value
|
Univariate analysis
P value
|
Multiivariate analysis
P value
|
GCS
|
r:0.687 P:0.012
|
0.012
|
0.097
|
CCT
|
r:-0.341 P:0.016
|
0.036
|
0.323
|
S100B
|
r:-0.710 P:0.003
|
0.002
|
0.009
|
GFAP
|
r:-0.699 P:0.002
|
0.003
|
0.303
|
Discussion
Initial assessment of the severity of head injury & immediate therapeutic measures are usually based on a combination of clinical examination & CCT. In numerous studies, it has been shown that this approach is effective, but far from perfect. Moreover, it remains difficult to assess secondary cellular damage inflicted by both primary trauma & secondary insults such as increased intracranial pressure or decreased cerebral perfusion. Similar to test for damage to other organs, a quick & simple test to quantitatively identify damage to the brain could be of great clinical value. Such a test could be based on the measurement of a biochemical neuromere. Ideally, such a neuromarker should be sensitive, highly brain specific, & measurable in blood rather than CSF. However, there is a lack of brain specificity of the most well-known neuromarker, hence we introduced a method for determination of GFAP & S100B from blood.
In this study, we examined 49 traumatic brain injury patients to compare the value of S100b & GFAP measurements in neurotrauma, analyzing whether these neuromarkers can yield additional prognostic information as compared to established measures of clinical examination & cranial computed tomography.
Clinical examination
To assess the neurologic status of the traumatic brain injury patients clinically, we used GCS. Several studies have shown a good correlation of the initial GCS score with early mortality & long - term morbidity in TBI patients [11]. Lately, however, these results have been questioned [5]. It needs to be considered that many traumatized patients are sedated & intubated immediately, which makes neurologic assessment difficult or even impossible. The results of our study are in accordance with the literature [17].
In our patients, the GCS score was significantly correlated with neurologic outcome. Severe head injury as indicated by a GCS score of 3-8 lead to unfavorable prognosis in 19 cases at the 6th month, whereas those with moderate head injury (GCS=9-12) lead to unfavorable prognosis in one case at the 6th month post injury.
The age of the patients has in most studies been found to be correlated with neurologic outcome after head trauma. Patients older than 65 years are especially at risk. In our study, mean values S100b & GFAP were higher in aged patients more than 60 years. The correlation between age & outcome was statistically not significant (P :> 0.05) at the 6th month. Absence of correlation may have attributed to less number of elderly patients in the study group.
Initial cranial computed axial tomography
It is undisputed that cranial computed axial tomography (CCT) on admission is a valuable tool in head trauma. Mass lesions requiring surgical therapy such as epidural or subdural hematomas are reliably recognized on CCT images. Other lesions, however, which can lead to severe neurologic damage such as diffuse axonal injury or lesions of brainstem are often not discernible on CCT images. That is why the prognostic value of initial CCT finding is lower than generally believed. Some CCT findings are undoubtedly associated with an unfavorable prognosis: Effacement of basal cisterns after head triples the mortality rate [13]. The presence of traumatic subarachnoid hemorrhage has been shown to double the mortality rate [12]. Cranial midline shifts by more than 5mm indicates an unfavorable outcome in 78% of patients older than 45 years. Yet, overall it is rather difficult to translate all findings on initial CCT into prognostic factor. Several scores have been proposed of which trauma coma data bank is commonly used. The disadvantage of this scale, it is not linear. It is obvious that an epidural hematoma, if evacuated timely may be associated with a better prognosis than severe diffuse injury with effacement of the basal cistern. Therefore, we analyzed patients with diffuse head injury separately from those with mass lesion. In doing so, we were able to show that mean values of S100b & GFAP levels were more in mass lesions in comparison to diffuse lesions, and it showed significant correlation (P:<0.05). CCT shows significant correlation with outcome (P≤ 0.05). On further analysis, outcome effect between diffuse (TCDB1-4) versus mass (TCDB5-6) lesion, did not show any significance (P:>0.05). So far, our results are in accordance with the literature [18].
Magnetic resonance image
Diffuse axonal trauma, damage to the corpus callosum, & brain stem injuries have been shown to indicate poor prognosis [19]. All these injuries can be visualized using magnetic resonance image (MRI), whereas they are often indiscernible on CCT scan. MRI is not available on 24h basis in our hospital. Undoubtedly, CCT is still the imaging modality of choice in acute neurotrauma. It has been proposed that MRI be performed within the first week after trauma to identify additional intra-axial brain damage [20].
S100B
In our study, the mean value of S100b is more in males, elderly patients, absent pupillary reaction, hypotensive, and hypoxic patients. The mean value of S100b in severe head injury (351.81) was more in comparison to moderate head injury (104.13). Mean S100b levels were more in mass lesions in comparison to diffuse lesions, but it did not show any significant correlation (P≤0.05). S100b is expressed not only in brain but also in extra cerebral tissues such as fat, skin, or muscle. Increased S100b levels are noted in patients undergoing heart surgery or extra cerebral trauma [15].
S100b levels positively correlated with injury severity score (r:0.346, P:0.15), hence it can be noted that S100b has an extra cranial source, and it is not a brain specific protein. This finding is in line with literature [17, 18]. Due to possibility of false positive results, it has been proposed that negative findings, i.e. low S100b levels are, of higher clinical significance than increased measurements.
S100B measurements showed the highest correlation with clinical outcome after head trauma & were effective to clinical assessment or CCT findings.
Glial fibrillary acidic protein (GFAP)
GFAP has advantage over S100b in that it seems to be specific for damage to CNS. Like S100b, it is released into the blood following head trauma. GFAP is a structural glial protein with higher molecular weight than that of the cytoplasmic protein s100b, and it is still not fully understood how GFAP is degraded in peripheral blood. Therefore, it is possible that the time course of GFAP blood concentrations after trauma is different from that of s100b.
The mean value of GFAP is more in males, elderly patients, absent pupillary reaction, hypotensive & hypoxic patients. The mean value of GFAP in severe head injury (5.61) was more in comparison to moderate injury (1.90). Mean GFAP levels were more in mass lesion in comparison to diffuse lesion, it was statistically significant (P≤0.05).
GFAP levels do not have correlation with injury severity score (r: 0.164, P: 0.259), hence it is noted that GFAP does not have an extra cranial source and it is a brain specific protein. We found that increased levels on admission were correlated with clinical outcome. Following initial reports, we have also reported increased levels in head trauma patients & found correlation with clinical outcome [17].
Conclusion
Our data showed that determination of serum S100b & GFAP level at the time of hospital admission has high rate of predicting outcome after traumatic brain injury when combined with clinical & CCT. The results of this study apply to the study population that satisfied the inclusion criteria. With respect to a possible clinical use of this method, this question is of high importance and should be addressed in future studies.
Conflicts of interest
Authors declare no conflicts of interest.
References
[1] Signorini DF, Andrews PJ, Jones PA, Wardlaw JM, Miller JD. Predicting survival using simple clinical variables: a case study in traumatic brain injury. J Neurol Neurosurg Psychiatry. 1999; 66(1):20–25.
[2] Bakay RA, Sweeney KM, Wood JH. Pathophysiology of cerebrospinal fluid in head injury: part 2. Biochemical markers for central nervous system trauma. Neurosurgery. 1986; 18(3):376–382.
[3] Bell MJ, Kochanek PM, Heyes MP, Wisniewski SR, Sinz EH, et al. Quinolinic acid in the cerebro-spinal fluid of children after traumatic brain injury. Crit Care Med. 1999; 27(3):493– 497.
[4] Hans P, Born JD, Chapelle JP, Milbouw G. Creatine kinase isoenzymes in severe head injury. J Neurosurg. 1983; 58(5):689–692.
[5] Kossmann T, Stahel PF, Lenzlinger PM, Redl H, Dubs RW, et al. Interleukin-8 released into the cerebrospinal fluid after brain injury is associated with blood-brain barrier dysfunction and nerve growth factor production. J Cereb Blood Flow Metab. 1997; 17(3):280–289.
[6] Morganti-Kossmann MC, Hans VH, Lenzlinger PM, Dubs R, Ludwig E, et al. TGF-beta is elevated in the CSF of patients with severe traumatic brain injuries and parallels blood-brain barrier function. J Neurotrauma. 1999; 16(7):617–628.
[7] Berger RP, Pierce MC, Wisniewski SR, Adelson PD, Clark RS, et al. Neuron-specific enolase and S100B in cerebrospinal fluid after severe traumatic brain injury in infants and children. Pediatrics. 2002; 109(2):E31.
[8] Kruse A, Cesarini KG, Bach FW, Persson L. Increases of neuron-specific enolase, S-100 protein, creatine kinase and creatine kinase BB isoenzyme in CSF following intraventricular catheter implantation. Acta Neurochir. 1991; 110(3-4):106–109.
[9] Hausmann R, Riess R, Fieguth A, Betz P. Immunohistochemical investigations on the course of astroglial GFAP expression following human brain injury. Int J Legal Med. 2000; 113(2):70–75.
[10] Herrmann M, Vos PE, Wunderlich MT, de Bruijn CH, Lamers KJB. Release of glial tissue-specific proteins after acute stroke: a comparative analysis of serum concentrations of protein S-100B and glial fibril-lary acidic protein. Stroke 2000; 31(11):2670–2677.
[11] Jiang JY, Gao GY, Li WP, Yu MK, Zhu C. Early indicators of prognosis in 846 cases of severe traumatic brain injury. J Neurotrauma. 2002; 19(7):869–874.
[12] Kakarieka A. Review on traumatic subarachnoid hemorrhage. Neurol Res. 1997; 19(3):230–232.
[13] Raabe A, Grolms C, Sorge O, Zimmermann M, Seifert V. Serum S-100B protein in severe head injury. Neurosurgery. 1999; 45(3):477–483.
[14] Yamazaki Y, Yada K, Morii S, Kitahara T, Ohwada T. Diagnostic significance of serum neuron-specific enolase and myelin basic protein assay in patients with acute head injury. Surg Neurol. 1995; 43(3):267–270.
[15] Missler U, Weismann M, Wittmann G, Magerkurth O, Hagenstrom H. Measurement of glial fibrillary acidic protein in human blood: analytical method and preliminary clinical results. Clin Chem. 1999; 45(1):138–141.
[16] van Geel WJ, de Reus HP, Nijzing H, Verbeek MM, Vos PE, et al. Measurement of glial fibrillary acidic protein in blood: an analytical method. Clin Chim Acta. 2002; 326(1-2):151–154.
[17] Vos PE, Lamers KJ, Hendriks JC, van Haaren M, Beems T, et al. Glial and neuronal protein in serum after severe traumatic brain injury. Neurology. 2004; 62(8):1303–1310.
[18] Wiesmann M, Steinmeier E, Magerkurth O, Linn J, Gottmann D, et al. Outcome prediction in traumatic brain injury: comparison of neurological status, CT findings, and blood levels of S100B and GFAP. Acta Neurol Scand. 2010; 121(3):178–185.
[19] Eisenberg HM, Gary HE Jr, Aldrich EF, Saydjari C, Turner B, et al. Initial CT findings in 753 patients with severe head injury. A report from the NIH Traumatic Coma Data Bank. J Neurosurg. 1990; 73(5):688–698.
[20] Firsching R, Woischneck D, Klein S, Ludwig K, Döhring W. Brain stem lesions after head injury. Neurol Res. 2002; 24(2):145–146.