Full Text
Introduction
Postural stability and stance dynamics are critical components of motor control, fundamental to the daily activities and overall physical health of children. These components are influenced by various factors, including age-related changes in foot biomechanics and the role of visual input in maintaining balance. Understanding these interactions is essential for developing interventions to improve postural stability and prevent injuries in paediatric populations.
Foot biomechanics, including parameters such as foot progression angle, arch index, and pressure distribution, undergo significant changes during childhood and adolescence [1–4].These changes can impact the way children maintain balance and distribute pressure across their feet, which in turn affects their postural stability and dynamic stance during movement. Visual input plays a pivotal role in maintaining postural stability, especially in children whose motor control systems are still developing. The visual cues significantly enhance balance and reduce sway in children, highlighting the importance of visual feedback in maintaining postural stability [5–7]. Various factors affecting plantar pressure have been studied including age and gender [1, 2]; obesity [8, 9]; foot deformities [8, 13] and diabetes [12]. Numerous studies have also examined the postural stability and plantar pressure parameters in healthy adults [13-15] and older adults [16]. However, the age-related alterations in plantar pressure among pre-adolescent and adolescent children within the Indian demographic have not been studied. Furthermore, the interaction between plantar pressure, visual input with postural stability and dynamic stance in the paediatric age group remains unexplored.
This study seeks to elucidate the differences between pre-adolescent (6-12 years) and adolescent (13-19 years) children by assessing parameters such as foot progression angle, arch index, and pressure distribution patterns. Additionally, the study aims to examine the impact of visual input on postural stability during static bipedal stance and dynamic stride tests, as well as interaction between age-related foot biomechanical changes and visual input on postural stability and stance dynamics to provide a comprehensive understanding of the developmental changes in motor control during these formative years.
Methodology
Sample size is calculated as 49 considering 2011 census and age wise population data till 2024, assuming the proportion of study population as 15% within 95% confidence limit and margin of error 10%.
Prospective observational study was conducted at Sanjay Ghodawat International School, Atigre, Maharashtra, India from March 2024 to April 2024. Participants were all residential students at the school, representing diverse regions across India. Informed assent was obtained from all participants and informed consent was obtained from their parents/legal guardians prior to their involvement in the study. Detailed information about the study objectives, procedures, potential risks, and benefits was provided to the participants and/or their parents/legal guardians. They were given the opportunity to ask questions before providing consent.
The study was conducted in accordance with the ethical standards of the 1964 Declaration of Helsinki and its later amendments. Ethical approval was granted by the Institutional ethics committee of Surya Hospital. Data confidentiality was maintained by anonymizing participant information and securely storing data in a restricted-access database.
Inclusion criteria: Children of both gender and irrespective of dominance aged 6 to 19 years of BMI within normal range as per age height and weight growth chart of IAP guidelines for Indian population [17].
Exclusion criteria: Individuals with any major musculoskeletal or neurological disorders affecting gait and posture, Individuals with significant visual impairments, history of recent lower limb injuries affecting gait, chronic conditions impacting foot biomechanics (e.g., severe flat feet, clubfoot).
The participants in the study were categorized into two groups: pre & early adolescent (6 to 12 years) and mid & late adolescent (13 to 19 years) [18]. The division of age groups was based on several considerations. Firstly, according to the World Health Organization (WHO) classification, adolescence is segmented in early mid & late stages. Additionally, the age of13 typically marks the onset of puberty and significant growth spurt, Demirbüken et al in their study highlighted the age 14 as critical age for alteration of plantar pressure [2]. Furthermore, the development of the longitudinal arch of foot can be identified till age of 12-13 years [4]. Also, most girls achieve final foot length at the age of 12 to 13 years, and boys from13-15 years [19]; making the age 13 years as a suitable sex-neutral boundary for a mixed small group of this study. Control variables included body mass index (BMI), with all participants having a normal BMI as per the age, height and weight growth chart of IAP guidelines for Indian population [17] and visual stimulus, ensuring that the study environment remained consistent for all participants. Dependent variables included several measures related to bipedal stance, stabilometry tests, and dynamic stride tests.
Data collection procedure
Anthropometric measurements
Height, weight, and BMI of each participant were recorded prior to the commencement of the testing session. Height was measured to the nearest centimetre using a stadiometer, weight was measured to the nearest kilogram using a calibrated scale and BMI was calculated using the standard formula (BMI = weight in kilograms / height in meters²).
Plantar pressure data collection was performed using the OHM 3000 portable plantar pressure system, a technology developed by Kan Innovations (https://www.kaninnovations.com/). This system operates on the principle of pedobarography [20, 21]. It features a sensor area of 380x 380 mm and contains 2916 sensors on a mat with dimensions of 701×508×8 mm, providing ample space for precise pressure measurements. The pressure data captured by these sensors is processed by the proprietary software (Ezra) to generate three-dimensional plots of pressure distribution; produce a pseudo-coloured map, schematic diagrams for deviation analysis, and visualizations of static pressure distribution. This data is further computed to give multiple ratios and averages. The following tests were conducted on each participant.
Bipedal stance test
Subjects were instructed to stand on the OHM 3000 mat with their feet a shoulder length apart. They were asked to keep their palms open and look straight ahead. The test duration was set to 20 seconds to capture stable bipedal stance data. The following parameters for both left and right feet were collected: maximum pressure, average pressure, weight distribution over the foot, foot area, foot length, forefoot width, hindfoot width, forefoot weight distribution, and hindfoot weight distribution.
Stabilometry test
Subjects were directed to stand on the pressure mat with their feet positioned as close together as possible. During the first trial, subjects maintained an eyes-open stance while looking straight ahead for 20 seconds. Subsequently, subjects repeated the test with their eyes closed to assess postural stability under reduced visual input conditions. The parameters collected were: weight distribution over the left and right feet, maximum pressure on both feet, weight distribution across forefoot and hindfoot regions, bilateral forefoot and hindfoot weight distribution, maximum lateral and anterior deviations, centre of pressure (COP) area for both feet, sway distance, and sway velocity.
Dynamic stance test
Subjects were instructed to walk comfortably on the pressure mat until they achieved a natural gait. Once the subject was walking with a normal gait pattern, data collection began. Data for both the right and left feet were recorded simultaneously to capture dynamic foot pressure distribution during walking. The test parameters collected for both feet included stance time, maximum pressure, average pressure, pressure peak, COP deviation, foot contact area, COP movement, loading, and off-loading slopes, centre of pressure excursion index, heel contact time, maximum heel pressure, midfoot contact time and maximum pressure, forefoot contact time and maximum pressure, hallux contact time and maximum pressure, and arch index.
Figure 1 demonstrates how the Ezra software visualizes data collected during the bipedal stance test, stabilometry test, and dynamic stance test. Note that this is a sample image and does not represent data from any specific subject in the study.
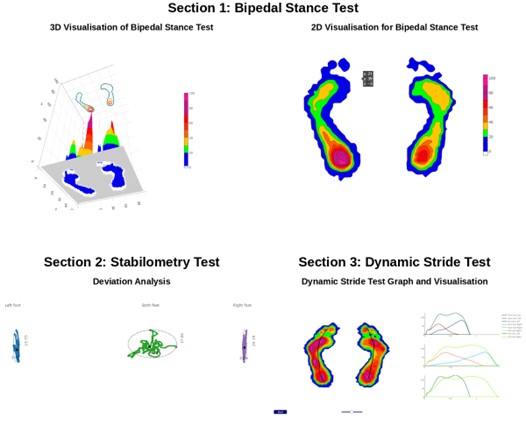
Figure 1: Sample visualization of plantar pressure data generated by the Ezra software.
Data analysis
Ezra, the proprietary software provided by Kan Innovations Inc., was used to extract the parameters in a tabulated format.
A two-tailed t-test was employed to analyse the relationships between independent and dependent variables a significance level of p < 0.05 (95% confidence) was used to determine the statistical significance of the t-tests.
Results
Total of 50 participants meeting the inclusion &exclusion criteria were a part of this study. Preadolescent group had 24 (48%) participants with mean age of 10.58±3.22 years (SD) and Adolescent group had 26 (52%) participants with mean age of 16.23±3.18 years (SD). The mean BMI of preadolescent group was 19.07 kg/m2as compared to 21.10 kg/m2 of adolescent group. The sex distribution between both groups was equivocal.
Bipedal stance: The bipedal stance test evaluates the static balance by analysing weight distribution and pressure in different age groups. The results are summarized in Table 1.
Table 1: Bipedal stance comparison.
|
Left
|
Right
|
Pre-adolescent (Mean ±SD)
|
Adolescent (Mean ±SD)
|
P<0.05
|
Pre-adolescent (Mean ±SD)
|
Adolescent (Mean ±SD)
|
P<0.05
|
Max pressure
|
64.77±23.19
|
81.50±22.24
|
0.0122
|
62.20±19.91
|
83.34±23.76
|
0.0014
|
Avg. pressure
|
15.53±1.77
|
19.37±2.95
|
0.0001
|
17.08±2.15
|
21.16±3.00
|
0.0001
|
Wt. distribution
|
48.65±8.25
|
47.47±4.72
|
0.5357
|
51.34±8.25
|
52.52±4.72
|
0.5357
|
Foot area
|
99.30±19.33
|
119.22±19.06
|
0.0006
|
95.36±20.75
|
120.79±18.60
|
0.0001
|
Foot length
|
23.08±1.92
|
24.84±1.68
|
0.0013
|
22.71±1.78
|
24.61±1.34
|
0.0001
|
Forefoot width
|
8.60±1.04
|
9.11±0.83
|
0.0622
|
8.47±0.99
|
9.13±0.88
|
0.0165
|
Hindfoot width
|
6.31±1.73
|
6.57±0.68
|
0.4895
|
6.26±0.91
|
6.76±0.73
|
0.0376
|
Forefoot weight distribution
|
34.17±8.30
|
33.82±9.78
|
0.8924
|
38.49±7.78
|
36.60±9.78
|
0.4558
|
Hindfoot weight distribution
|
65.82±8.30
|
66.17±9.78
|
0.8924
|
61.50±7.78
|
63.39±9.78
|
0.4558
|
Note: Statistically significant difference in maximum pressure, average pressure, foot area and foot length are exhibited in both feet with higher values in adolescents as compared to pre-adolescents.
Difference between forefoot and hindfoot width is not significant on left side but on right side significant difference is observed due to smaller right foot in preadolescent and larger right foot in adolescent.
No difference was noted regarding weight distribution over the whole foot, fore foot or hind foot on both feet when adolescents were compared to pre-adolescents despite changes in foot size and pressure.
Stabilometry test
The stabilometry test with eyes open (EO) and eyes closed (EC) evaluates postural control and balance under different sensory conditions due to visual inputs. The parameters for the stabilometry test are compared in Table 2 and 3.
Table 2: Stabilometry test comparison between left and right foot when eyes are open and closed.
Eyes Open (EO)
|
|
Left
|
Right
|
Pre-adolescent (Mean ±SD)
|
Adolescent (Mean ±SD)
|
P<0.05
|
Pre-adolescent (Mean ±SD)
|
Adolescent (Mean ±SD)
|
P<0.05
|
Weight distribution
|
49.34±7.11
|
50.29±5.34
|
0.5947
|
50.65±7.11
|
49.70±5.34
|
0.5947
|
Max pressure
|
99.38±0.18
|
112.60±31.32
|
0.1859
|
88.55±33.99
|
101.18±26.15
|
0.1454
|
Weight distribution - forefoot
|
38.61±9.17
|
41.35± 14.36
|
0.4294
|
43.11± 8.88
|
44.32±12.25
|
0.6943
|
Weight distribution -hindfoot
|
61.38±9.17
|
58.64± 14.36
|
0.4294
|
56.88±8.88
|
55.67±12.25
|
0.6943
|
Max lateral deviation
|
10.08±5.24
|
8.78±4.40
|
0.3454
|
10.42±4.02
|
7.76±3.49
|
0.0156
|
Max anterior deviation
|
18.35±9.12
|
15.88± 7.25
|
0.2923
|
19.29±9.14
|
15.53±7.61
|
0.1201
|
Max posterior deviation
|
21.9±12.35
|
16.10± 4.58
|
0.0279
|
20.35±9.65
|
15.83±6.90
|
0.0612
|
COP area
|
250.09 ±183.82
|
176.7±153.10
|
0.1305
|
233.11±133.75
|
167.57±141.25
|
0.0992
|
Eyes closed (EC)
|
Weight distribution
|
49.66±7.35
|
50.58±4.59
|
0.5982
|
50.34±7.35
|
49.42±4.59
|
0.5982
|
Max pressure
|
105.11±39.95
|
112.85±31.08
|
0.4459
|
94.59±32.09
|
101.88±25.1
|
0.3739
|
Weight distribution - forefoot
|
38.09±8.87
|
43.64± 12.16
|
0.0732
|
42.56±9.3
|
47.14±10.59
|
0.1125
|
Weight distribution - hindfoot
|
61.91±8.87
|
56.36± 12.16
|
0.0732
|
57.44±9.3
|
52.86±10.59
|
0.1125
|
Max lateral deviation
|
10.52±4.25
|
11.17±5.49
|
0.6418
|
11.05±4.07
|
9.8±3.87
|
0.2686
|
Max anterior deviation
|
19.35±6.86
|
17.23±5.77
|
0.2416
|
20.26±7.72
|
18.22±6.17
|
0.3051
|
Max posterior deviation
|
25.32±13.24
|
18.95±7.34
|
0.0386
|
21.04±11.23
|
21.17±8.77
|
0.965
|
COP area
|
293.09 ±195.59
|
254.61±152.04
|
0.4392
|
294.27±221.6
|
240.79 ±135.68
|
0.3044
|
Maximum pressure and weight distribution over whole feet, fore foot, hind foot was not different significantly on both right and left foot during eyes open as well as eye closed.
There was no significant difference in anterior deviation and centre of pressure among both groups in both side in eye open as well closed. But maximum lateral deviation was significantly less in adolescent with eyes open toward right feet as compare to preadolescents and the difference was lost with eyes closed. While significantly less posterior deviation was observed in adolescents during eye open as well as closed in left foot as compare to preadolescents.
Table 3: Stabilometry test comparison of both feet together when eyes are open and closed.
|
EO both feet
|
EC both feet
|
Pre-adolescent (Mean ±SD)
|
Adolescent (Mean ±SD)
|
P < 0.05
|
Pre-adolescent (Mean ±SD)
|
Adolescent (Mean ±SD)
|
P< 0.05
|
B/L weight distribution Forefoot
|
40.74±8.54
|
42.70±12.72
|
0.5303
|
40.75±8.55
|
45.20±10.76
|
0.1143
|
B/L weight distribution hindfoot
|
59.25± 8.54
|
57.29±12.72
|
0.5303
|
59.24±8.55
|
54.79±10.76
|
0.1433
|
Max lateral deviation
|
34.59±8.38
|
26.84±10.14
|
0.0052
|
41.16±11.72
|
36.99± 9.71
|
0.1758
|
Max anterior deviation
|
16.06±8.61
|
13.57±5.71
|
0.2314
|
16.80 ±5.79
|
15.56±5.06
|
0.4214
|
Max posterior deviation
|
15.67±8.11
|
13.53±4.21
|
0.2427
|
17.64± 8.07
|
16.16±6.81
|
0.4858
|
COP area
|
724.51±402.87
|
493.82± 329.69
|
0.0310
|
952.14±512.6
|
782.95±344.94
|
0.1743
|
Sway distance
|
380.51± 95.49
|
312.33±94.45
|
0.0145
|
475.81 ±124.01
|
437.25±129.37
|
0.2883
|
Sway velocity
|
19.3±4.85
|
15.90±4.79
|
0.0143
|
24.23±6.28
|
22.27±6.59
|
0.2882
|
Considering both feet together for stabilometry, there is no statistically significant difference among weight distribution, maximum anterior deviation and maximum posterior deviation among both eyes open as well as closed condition.
There is statistically significant difference with less lateral deviation, condensed centre of pressure area, and less sway distance and less sway velocity when eyes open and visual input is present. All this difference is lost when eyes were closed in adolescents as compare to preadolescents.
Dynamic stride test
The dynamic stride test assesses gait parameters and pressure distribution during walking. The parameters of the dynamic stride test are compared in Table 4.
Table 4: Dynamic stride test comparison between left and right foot.
|
Left
|
Right
|
Parameters
|
Pre-adolescent
(Mean ±SD)
|
Adolescent
(Mean ±SD)
|
p values of
t-test
|
Pre-adolescent
(Mean ±SD)
|
Adolescent
(Mean ±SD)
|
p values
of t-test
|
Stance time
|
0.97±0.17
|
1.01±0.23
|
0.4838
|
0.98±0.18
|
1±0.13
|
0.6887
|
Max pressure
|
178.88±43.36
|
212.78±46.92
|
0.011
|
175.82±44.94
|
231.83±91.78
|
0.0095
|
Average pressure
|
22.89±2.6
|
27.75±3.78
|
0.0001
|
22.06±2.37
|
26.64±3.15
|
0.0001
|
Pressure peak time
|
0.76±0.19
|
0.78±0.28
|
0.8181
|
0.67±0.25
|
0.73±0.18
|
0.3317
|
COP deviation
|
1.9±0.66
|
2.06±0.62
|
0.3623
|
2.14±0.52
|
2.19±0.68
|
0.737
|
Foot contact area
|
149.36±20.99
|
174.96±20.72
|
0.0001
|
150.73±19.09
|
172.2±16.7
|
0.0001
|
Loading slope
|
238.42±132.81
|
281.85±126.64
|
0.2424
|
275.79±179.64
|
304.15±162.63
|
0.5606
|
Offloading slope
|
904.83±380.88
|
916.58±397.97
|
0.9157
|
956.92±706.67
|
1120.04±637.29
|
0.395
|
Center of pressure
|
17.85±10.92
|
17.41±9.14
|
0.8767
|
15.76±9.48
|
16.46±9.59
|
0.796
|
Heel contact time
|
0.67±0.15
|
0.7±0.22
|
0.5534
|
0.62±0.16
|
0.66±0.16
|
0.3408
|
Maximum pressure
at heel
|
130.8±43.56
|
154.95±154.54
|
0.465
|
131.05±32.72
|
149.13±38.36
|
0.0805
|
Midfoot contact time
|
0.8±0.14
|
0.82±0.13
|
0.6899
|
0.77±0.18
|
0.84±0.12
|
0.0977
|
Midfoot max pressure
|
82.68±39.77
|
109.39±50.42
|
0.044
|
95.47±46.12
|
134.86±105.84
|
0.0993
|
Forefoot contact time
|
0.91±0.14
|
0.92±0.11
|
0.8633
|
0.91±0.16
|
0.95±0.13
|
0.3678
|
Forefoot - max
pressure
|
174.31±42.78
|
212.1±47.74
|
0.0051
|
172.93 ±46.61
|
220.79 ±63.51
|
0.0041
|
Hallux contact time
|
0.73±0.17
|
0.77±0.15
|
0.4391
|
0.67±0.18
|
0.77±0.18
|
0.0559
|
Hallux –
max pressure
|
67.24±39.21
|
88.52±41.01
|
0.0674
|
39.26±16.32
|
55.72±37.22
|
0.0517
|
Arch index
|
0.26±0.03
|
0.27±0.03
|
0.4443
|
0.26±0.03
|
0.26±0.03
|
0.7247
|
There is statistical significance in maximum pressure, average pressure, foot contact area and forefoot maximum pressure in both feet. While other parameters were not different indicating consistent gait cycle phases across age groups.
Discussion
In various studies, the reliability of plantar pressure measurement systems has been extensively evaluated [22-24]. Sawant and Vaidya (2022) found that the OHM 3000 system demonstrated high reliability for both static and dynamic pressure assessments in a healthy Indian population. Their study, involving 28 participants, measured key variables such as maximum pressure, average pressure, contact area, and weight distribution [23].
In both genders, age significantly influences plantar pressure distribution and maximum pressure, especially during early adolescence showing significantly higher values compared to younger ages. Understanding age-related changes in postural stability and dynamic stance is essential for developmental biomechanics [2].
A cross-sectional study on children with autism spectrum disorder (ASD) emphasizing the need for reliable measurement systems when evaluating postural stability in paediatric populations. The study highlighted the importance of foot morphology to ensure accurate and reliable postural stability assessments [26].
Visual input significantly affects postural stability [7, 25]. Research has highlighted the importance of including visual conditions in postural stability assessments to fully understand stability dynamics and for designing interventions [26].
In our study, adolescents were having significant higher maximum pressure, average pressure, foot area and foot length in both feet, indicating an increase in foot load likely due to greater body weight and muscle development in adolescents, consistent with overall growth patterns during adolescence.
Significant difference due to smaller right foot in preadolescent and larger right foot in adolescent is observed in forefoot and hindfoot width that may be due to right dominance affecting the growth of right foot.
No difference in weight distribution despite change in foot size and foot pressure was observed. It may be in line with consistent foot pressure distribution biomechanics.
For postural stability, maximum pressure and weight distribution was not different in both foot as well as individual foot with eye open as well as eye closed. It may signify that these parameters are consistent with minimal visual input. These trends suggest that even without visual input, adolescents handle higher pressures due to physical growth.
Maximum lateral deviation was significantly less in right foot as well as both feet when eyes are open but the difference is lost when eyes were closed. Visual input play is very important role to minimize the lateral deviation and right preponderance may be seen as children grow, indicating possible adaptations in gait and balance with age.
Maximum posterior deviation is significantly less in adolescent as compare to preadolescents in both open and closed eyes towards left foot. It may denote the stronger balancing for less posterior deviation in adolescent regardless of visual input.
Considering the balance in both feet together, visual input plays importantly to maintain postural balance in adolescents as we observed reduced lateral deviation, COP area, sway distance and sway velocity with open eye in adolescents indicating improved postural stability.
These results indicate that postural stability is superior in the older age group regardless of the visual input. The increased foot length, area, and pressure possibly contribute to this enhanced stability. This suggests that the foot maturation occurring during adolescence plays a significant role in improving postural stability.
During walking, gait analysis and dynamic stride test revealed, no difference in stance time, peak pressure time, COP & COP deviation, loading and offloading slope, Arch Index and contact time of all areas of foot indicating stable foot biomechanics during development in view of statistically raised maximum pressure, average pressure, foot contact area in adolescent. Raised maximum pressure in mid foot and forefoot is significantly more in adolescent as compare to preadolescent, probably due to more pressure bearing of forefoot as compare to heel during walking with advancing age.
The study's strengths include its focus on the Indian demographic, providing specific insights relevant to this population, comprehensive data collection using advanced technology, and a wide age range allowing a thorough analysis of developmental changes.
However, limitations include small sample size, cross-sectional design, and single site for data collection, which restrict generalizability. Further research opportunities include developing targeted interventions for improving postural stability and expanding research to diverse populations and settings. Potential threats include unaccounted confounding variables affecting results and technological limitations in measuring certain dynamic aspects of gait.
Recommendations for future studies include conducting longitudinal studies to establish causal relationships between age-related changes in foot biomechanics and postural stability, increasing sample size to enhance robustness, implementing multi centred studies to provide comprehensive insights across different regions, and exploring intervention studies to develop effective strategies for improving postural stability in children.
Conclusion
The present study offers significant insights into the age-related alterations in foot biomechanics. Key outcomes include increased maximum pressure, average pressure, foot area and foot length indicating physical growth and higher load-bearing capacities in adolescents compared to pre-adolescents. Despite significant changes in foot size and pressure, weight distribution over the forefoot and hindfoot remained consistent across age groups, suggesting a robust adaptation mechanism that maintains balance despite physical growth. Visual input significantly improved postural stability in both groups. Postural stability was better in adolescents irrespective of the visual input probably due to foot maturation. Although visual input is especially important to reduce lateral deviation, COP area, sway distance and sway velocity. During walking, Adolescents showed higher pressure handling capacities with increased maximum and average pressures, especially in forefoot. Other parameters of dynamic stride like stance time, foot progression angle and loading and offloading slopes remained stable, indicating consistent gait parameters across age groups.
Acknowledgment
Mr. Kunal Dattir, Ms. Sasmita Mohanty and the staff of Sanjay Ghodawat International School.
Conflicts of interest
Authors declare no conflicts of interest.
References
[1] Machado ÁS, Bombach GD, Duysens J, Carpes FP. Differences in foot sensitivity and plantar pressure between young adults and elderly. Arch Gerontol Geriatr. 2016; 63:67–71.
[2] Demirbüken İ, Özgül B, Timurtaş E, Yurdalan SU, Çekin MD, et al. Gender and age impact on plantar pressure distribution in early adolescence. ActaOrthopTraumatolTurc. 2019; 53:215–220.
[3] Anderson M, Blais M, Green WT. Growth of the normal foot during childhood and adolescence. Length of the foot and interrelations of foot, stature, and lower extremity as seen in serial records of children between 1–18 years of age.Am J Phys Anthropol. 1956; 14:287–308.
[4] Fritz B, Mauch M. Foot development in childhood and adolescence. Handbook of footwear design and manufacture, Elsevier Inc.; 2013, pp. 49–71.
[5] Ghorbani M, Yaali R, Sadeghi H, Luczak T. The effect of foot posture on static balance, ankle and knee proprioception in 18-to-25-year-old female student: a cross-sectional study. BMC Musculoskelet Disord. 2023; 24:547.
[6] Mergner T, Schweigart G, Maurer C, Blümle A. Human postural responses to motion of real and virtual visual environments under different support base conditions. Exp Brain Res. 2005; 167:535–556.
[7] Sparto PJ, Redfern MS, Jasko JG, Casselbrant ML, Mandel EM, et al. The influence of dynamic visual cues for postural control in children aged 7-12 years. Exp Brain Res. 2006; 168:505–516.
[8] Yan S, Li R, Shi B, Wang R, Yang L. Mixed factors affecting plantar pressures and center of pressure in obese children: Obesity and flatfoot. Gait Posture. 2020; 80:7–13.
[9] Dowling AM, Steele JR, Baur LA. Does obesity influence foot structure and plantar pressure patterns in prepubescent children? Int J Obes Relat Metab Disord. 2001; 25:845–852.
[10] Khalaf K, Mohan DM, Hindi M Al, Khandoker AH, Jelinek HF. Plantar pressure alterations associated with increased BMI in young adults. Gait Posture. 2022; 98:255–260.
[11] Pauk J, Daunoravičienė K, Ihnatouski M, Griskevicius J, Daunoraviciene K, et al. Analysis of the plantar pressure distribution in children with foot deformities Analysis of the plantar pressure distribution in children with foot deformities. 2010;12.
[12] Chatwin KE, Abbott CA, Boulton AJM, Bowling FL, Reeves ND. The role of foot pressure measurement in the prediction and prevention of diabetic foot ulceration-A comprehensive review. Diabetes Metab Res Rev. 2020; 36:e3258.
[13] De Blasiis P, Caravaggi P, FullinA, Leardini A, Lucariello A, et al. Postural stability and plantar pressure parameters in healthy subjects: variability, correlation analysis and differences under open and closed eye conditions. Front Bioeng Biotechnol. 2023; 11.
[14] Mun KR, Chun S, Hong J, Kim J. The relationship between foot feature parameters and postural stability in healthy subjects. Hum Factors. 2019; 61:1077–1085.
[15] Boukhenous S, Attari M. A postural stability analysis by using plantar pressure measurements.Eighth international multi-conference on systems, signals & devices, IEEE; 2011; pp.1–6.
[16] Unver B, Bek N. Plantar sensation, plantar pressure, and postural stability alterations and effects of visual status in older adults. Somatosens Mot Res. 2022; 39:55–61.
[17] Khadilkar V, Yadav S, Agrawal KK, Tamboli S, Banerjee M, et al. Revised IAP growth charts for height, weight and body mass index for 5-to 18-year-old Indian children. 2015; 52:47–55.
[18] de Onis M, Garza C, Onyango AW, Rolland-Cachera MF. WHO growth standards for infants and young children. Archives de Pediatrie. 2009; 16:47–53.
[19] Cheng JC, Leung SS, Leung AK, Guo X, Sher A, et al. Change of foot size with weightbearing.A study of 2829 children 3 to 18 years of age. Clin Orthop Relat Res. 1997:123–231.
[20] Lorkowski J, Gawronska K, Pokorski M. Pedobarography. A review on methods and practical use in foot disorders. Applied Sciences. 2021; 11.
[21] Skopljak A, Muftic M, Sukalo A, Masic I, Zunic L. Pedobarography in diagnosis and clinical application. Acta Informatica Medica. 2014; 22:374–378.
[22] Martínez-Nova A, Cuevas-García JC, Pascual-Huerta J, Sánchez-Rodríguez R. BioFoot® in-shoe system: Normal values and assessment of the reliability and repeatability. Foot. 2007; 17:190–196.
[23] Sawant N, Vaidya S. Reliability of OHM 3000 plantar pressure system for measure-ment of plantar pressures in healthy Indian population. Int J Physioth Res. 2022; 10:4095–4101.
[24] Gurney JK, Kersting UG, Rosenbaum D. Between-day reliability of repeated plantar pressure distribution measurements in a normal population. Gait & Posture. 2008; 27:706–709.
[25] Reimann H, Ramadan R, Fettrow T, Hafer JF, Geyer H, et al. Interactions between different age-related factors affecting balance control in walking. Front Sports Act Living. 2020; 2.
[26] Li Y, Liu T, Venuti CE. Development of postural stability in children with autism spectrum disorder: a cross-sectional study. Int Biomech. 2021; 8:54–62.